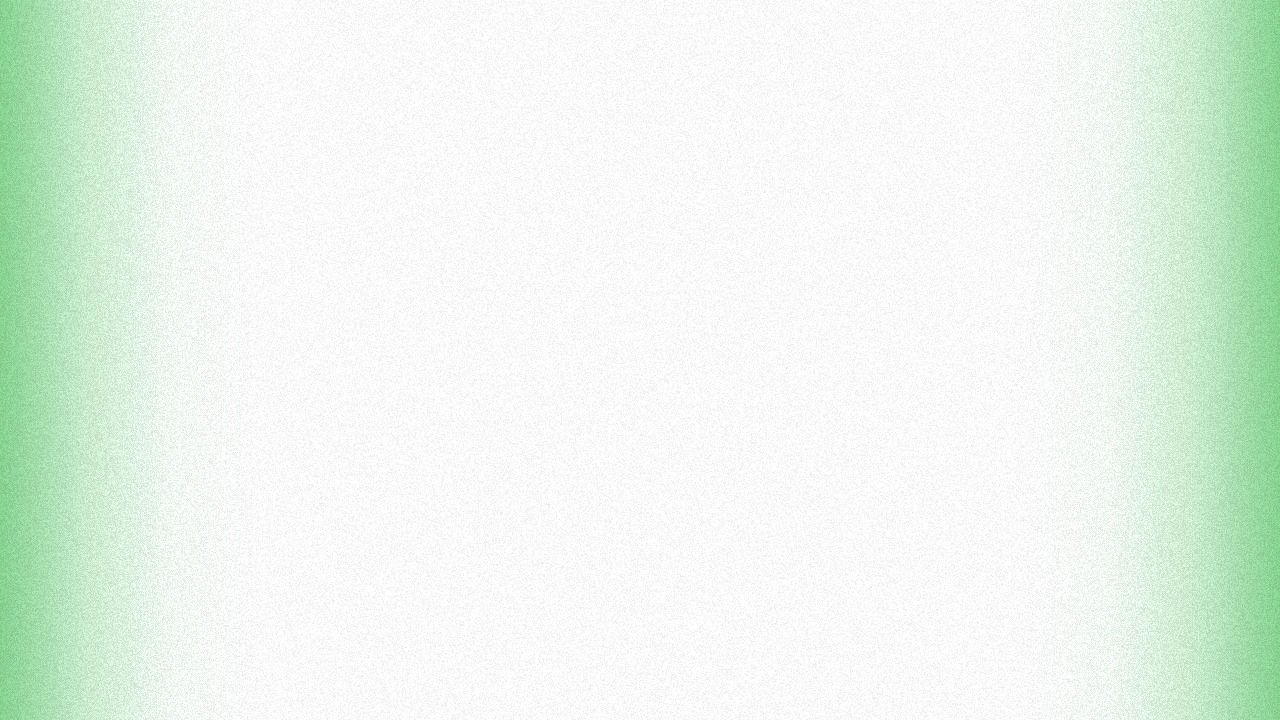
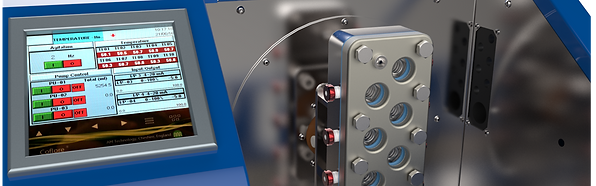
This site uses cookies. Click here to find out more.
A Batch Energy Consumption Comparison
100 L Coflore RTR vs. 13.6 m³ Batch Reactor
Introduction
The Coflore Rotating Tube Reactor (RTR) has a 100 L capacity that is capable of processing countless reactor volumes without interruption. For a 10-minute residence time, the RTR can generate 14.4 m³ of material within a 24-hour period. The productivity of an RTR is comparable to a 3000 imperial gallon (13.6 m³) batch reactor performing one batch per day.
The following will calculate and illustrate possible power savings with respect to heating, cooling, and electrical demand for a common anonymized chemical reaction.
Summary
Even without a heat integration network, the RTR requires 27 times less peak heating and 5.9 times less peak cooling than an equivalent batch process. On top of the reduction in utility requirements, the RTR requires less operating volume than the equivalent batch reactor. This can lead to additional energy savings such as reduced HVAC energy consumption by operating in a smaller building.
Assumptions
-
Heating is supplied by 3 BarG steam with condensing jackets
-
Cooling water supplied at 10 °C and is raised to a maximum of 40 °C by cooling processes
-
The frame of reference for utility requirements is with regards to the heat transfer streams (-X kW implies heating, +Y kW implies cooling)
-
A 3000 gallon batch vessel has a heat transfer surface area of 25.2 m² when filled to the nominal capacity
-
The heat transfer surface area decreases linearly with fill percentage.
-
Heat transfer coefficients of 316 W/m² K and 185 W/m² K have been taken for heating and cooling processes, respectively
-
It was assumed that no heat losses occur in either batch or flow
-
It was assumed that heat capacity is invariant with respect to temperature
-
No enthalpies of mixing were accounted for
-
Total volume and enthalpy were assumed to be a sum of pure component volumes and enthalpies
-
Electrical power demand is based on the motor rating rather than the real load
-
No margins of error have been applied
Estimation of Heating, Cooling, and Electrical requirements in Batch
For the batch process, the sequence can be broken down into 6 steps. Both the peak and average utility requirements have been calculated for each step in the sequence (Table 1).
-
Step 1: Heating of reagent A in water from 10 °C (storage temperature) to 80 °C
-
Step 2: Dropwise addition of reagent B to the reactor. Reagent B is added at 10 °C and instantaneously reacts exothermically. The reactor temperature of 80 °C is maintained during the addition
-
Step 3: Cooling of the reactor vessel contents from 80 °C to 25 °C
-
Step 4: Heating of an aqueous CIP fluid from 10 °C to 80 °C
-
Step 5: Reactor contents are stirred for 2 hours with no change in temperature or reaction
-
Step 6: Cooling of the reactor vessel contents from 80 °C to 25 °C
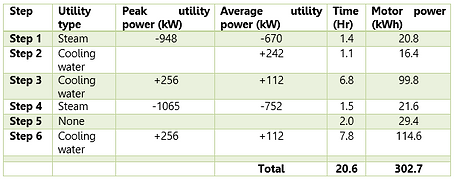
Table 1: Utility Demand Breakdown for each Step in the Batch Sequence
The six process steps outlined require a total time of 20.6 hours based on the assumptions laid out. This allows for 3.4 hours in the batch sequence for fluid transfers, draining of the jacket when changing utility media, as well as a time contingent.
Estimation of Heating, Cooling, and Electrical requirements in the RTR
In the RTR, a residence time of 10 minutes will produce 14.4 m³ of product within a 24-hour period. In order to convert the same reaction performed in batch to continuous production;
-
Reagent A in water is continuously fed into the RTR at 80 °C
-
Reagent B is continuously fed into the RTR at 10 °C
-
The reactor is supplied with cooling water onto the jacket and the process temperature at the outlet is maintained at 80 °C
-12.8 kW (heating) are generated from the heats of reactions. -1.9 kW (heating) and +2.3 kW (cooling) are generated from the enthalpic change in the compounds and raising the temperature of reagent B, respectively. In total +12.4 kW (cooling) is required to the RTR to maintain the outlet temperature at 80 °C. To run the RTR for 24 hours, there is an electrical power demand of 52.8 kWh.
To allow for a fair comparison, it should be stated that -41.8 kW of heating are required to heat the stream reagent A in water from 10 °C to 80 °C prior to addition to the RTR. +33.3 kW of cooling are required to cool the reactor product stream from 80 °C to 25 °C.
Since the RTR is a continuous flow reactor, the net utility requirements can be further reduced with heat integration circuits. By using the warmed cooling water from the reactor, and the reactor product stream, the reactor feed can be partially heated. By taking the total cooling demand of +45.7 kW, and the total heating demand of -41.8 kW, the theoretical minimum net utility demand is +3.9 kW of cooling. Reaching the theoretical minimum net utility demand is inherently impossible but a well-designed heat integration network can provide significant savings.
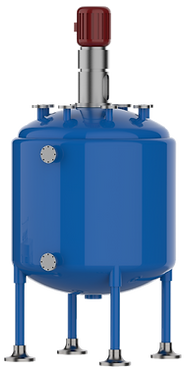
Nominal Size comparison between Coflore RTR vs 3000 (13.6 m³) Gallon Batch Reactor

Summary Comparison
Table 2 shows the calculated summary of utility requirements for both the 3000 gallon batch reactor as well as the 100 litre RTR. It can be seen that there are significant reductions in demand even without a heat integration network.
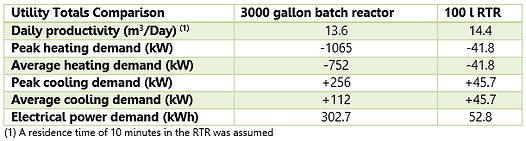
Table 2: Summary of Utility Requirements for each reactor
Since the daily productivities shown in Table 2 are not equal, it is necessary to normalise the utility requirements to the daily productivity for each reactor. Table 3 shows the normalised utility requirements as well as the reductions ratio for scaling out a process.
On top of the reduction in utility requirements, the RTR required less operating volume than the equivalent batch reactor. This can lead to additional energy savings such as reduced HVAC energy consumption by operating in a smaller building.
Even without a heat integration network, the RTR requires 27 times less peak heating and 5.9 times less peak cooling than an equivalent batch process.
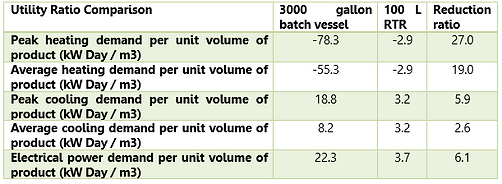
Table 3: Normalised summary of Utility Requirements for each Reactor